Probable or Definite?
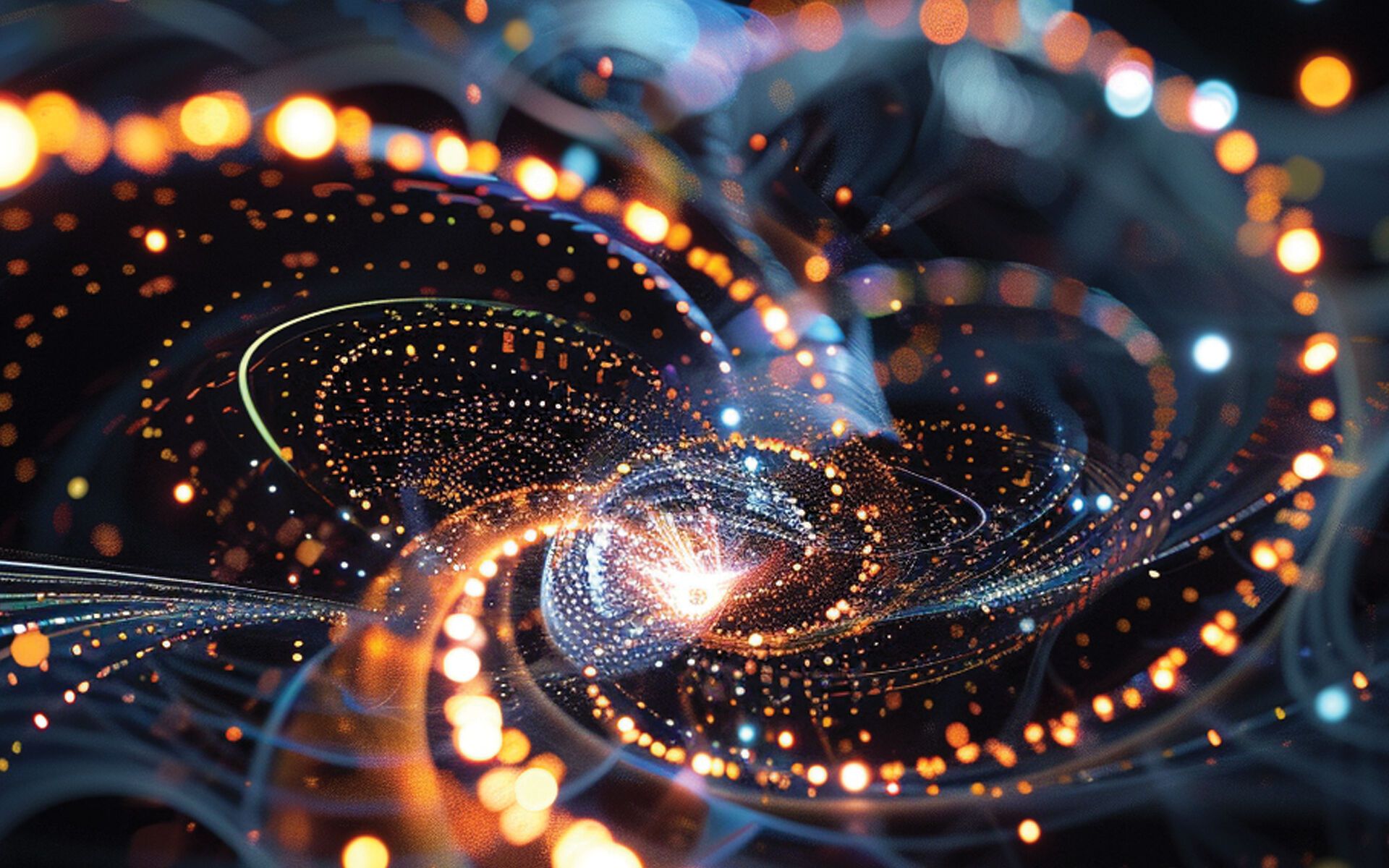
In This Article
-
The certainty in Classical Physics fades away within the realm of the quantum mechanics, not due to limitations in our knowledge or measurement capabilities, but as an intrinsic quality of quantum entities.
-
Before we make an observation, a quantum particle or system is in a superposition state lacking well-defined properties that preclude assertions about its physical attributes. The particle manifests a specific property only after an observation.
Physics is one major scientific discipline that helps us understand the universe. While classical Physics is more about the world and things we can observe, we study the matter at the atomic and subatomic level with quantum physics. Quantum physics (or quantum mechanics) is best known as probabilistic as opposed to being deterministic. Classical Physics, or any branch of science for that matter, is deterministic in the sense that we can predict the results of an experiment accurately provided we have all the available information. For instance, when throwing a ball into the air, I can, with certainty, predict the trajectory of the ball if factors such as initial speed, wind velocity, and air resistance are known. However, this certainty fades away within the realm of quantum mechanics, not due to limitations in our knowledge or measurement capabilities, but as an intrinsic quality of quantum entities.
Subatomic particles, referred to as quantum objects, include entities like protons, electrons, and atoms. While quantum mechanics’ principles theoretically extend to sizable objects, their effects become imperceptible, primarily owing to a phenomenon physicists call decoherence. Decoherence emerges from interactions with the many particles in the environment, effectively erasing quantum behavior, a process likened to the “washing out” of quantum features. If we could isolate an atom perfectly from its surroundings, we would be able to see quantum behavior; but in everyday large objects there exist a huge number of particles which renders the observation of quantum behavior practically impossible.
To begin, let’s delve into the concept of probability. In the realm of quantum mechanics (QM), a particle has the potential to exist in a linear combination or superposition of multiple states. To simplify and sidestep technical jargon, let’s consider the “color” property of a quantum object, such as an electron. While electrons don’t possess color in the conventional sense, the analogy applies to measurable physical attributes like position, velocity, or spin. When measured, the color of an electron is always either a red or a blue. Classical logic asserts that an object’s color is an unchanging, fixed property, unaffected by observation. However, QM challenges this notion, proposing that the object exists as a blend of red and blue until subjected to observation or measurement. There is a certain probability of getting red, and getting blue; however, we cannot tell which one. Let’s assume these probabilities are 50% each. This would mean if I measured the color of an electron, I would get red or blue with a 50% probability for each. The math of QM can calculate the probabilities with accuracy but dictates that the color was in a superposition state going back and forth between the two prior to observation. Suppose the measurement reveals the color red. The intriguing question then arises: “Was it red before the observation?” Although specific probabilities are linked to each color in an observation, certainty about the revealed color remains mysterious. The act of observation leads to the phenomenon termed the “collapse of the state,” signifying the transformation from a two-state mixture (superposition) to a definitive state of either red or blue.
The renowned double-slit experiment is a typical illustration demonstrating the concept of superposition. Here, we will provide a concise description of the double slit experiment without delving into technicalities. Imagine sending electrons through a double-slit configuration one at a time. Once through, the electron strikes a screen positioned beyond the slits. Each electron leaves a mark on the screen like a point particle. As we send more electrons, one at a time, a pattern gradually builds up on the screen generated by the many marks left by the electrons. The pattern is exactly a copy of what a wave would do. So, the outcome is that the electrons collectively behave as a wave – much like a water wave that would pass through both slits simultaneously and hit the screen. This is what physicists call wave-particle duality: when an electron behaves like a particle as it leaves a point mark on the screen, but the collective behavior is one of waves (not many particles). To explain the wavy pattern formed at the screen, we are compelled to accept that the electron is just like a wave passing through the two slits simultaneously. In the language of QM, the electron is in a superposition state of being at both slits at the same time. Things get even more interesting once we devise an experiment to measure which slit it “really” goes through. Experiments like this have undoubtedly confirmed that if we have the “which slit” information, the superposition state collapses: the electrons are observed to pass through one of the two slits and, moreover, they form a pattern on the screen that particles would generate. The overall inference is that until we observe which slit an electron goes through, we must treat the electron as being in a state of superposition, i.e. going through both slits simultaneously. However, an observation will make it collapse to a state of passing through only one slit.
The mathematical framework was clear: before we make an observation, a quantum particle or system is in a superposition state lacking well-defined properties that preclude assertions about its physical attributes. The particle manifests a specific property only after an observation. This quantum behavior has been confirmed countless times especially with experiments involving electron spins and photons (light particles). In summary, QM essentially argues that every tiny particle is in a mixed state consisting of all possible realities until subjected to observation. Since every entity, living or nonliving, is made up of tiny quantum particles like protons, electrons and neutrons, this should apply to any object in the universe. It is the act of observation that brings out the reality; no inherent “real property” existed prior to measurement.
During the initial stages of its development, the idea of superposition was taken with skepticism notably by the famous Albert Einstein. Einstein acknowledged that the observations are explained by this new weird mathematical framework of QM; however, he believed there is more to unravel. Einstein basically argued that a particle must always have a definite property even before the measurement. To him, the notion that a particle lacked a “real” value in the absence of observation seemed illogical. Einstein is reported to have said, “I would like to think that the Moon is still there even if I do not look at it.” This stance aligns with the concepts of “localism” and “realism,” suggesting a persistent, definite reality irrespective of observation.
At this juncture, the issue becomes more philosophical than scientific. The pivotal question emerges: did the electron have a real and definite color prior to observation? (In lieu of color, the experiments would measure physical properties like spin or position). QM will respond “no, it exists in a superposition state.” In contrast, classical logic or Einsteinian viewpoints would say “yes, of course! It has always had a definite color; we just did not know it.” This debate is deeply related to measurement theory as well. To put it from a different standpoint, measurement in classical logic discloses an object’s property, while in QM, measurement forces the object to assume one of its potential and observable properties.
In opposition to this idea of QM, Einstein and his colleagues published the renowned EPR paper titled “Can Quantum Mechanical Description of Physical Reality Be Considered Complete?” In this article, Einstein argued that even though the Math of QM is accurate, QM is insufficient in addressing the measurement problem. The authors basically argued that there is more to the story and postulated the existence of “hidden variables” (HV). According to this perspective, these hidden variables serve as the “genes” of a particle, akin to an inaccessible genetic code. Though beyond our reach, the realist viewpoint, or the HV theory, asserts their existence, containing information about a particle’s measurement outcome. Take, for instance, the spin property of an electron, which can be up or down when measured along a certain axis. If not observed, the electron exists in a superposition/mixed state of up and down according to QM principles. Only upon observation, it takes on either one of the values (up or down); and we cannot know which one. In contrast, the EPR paper proposes that the particle has a HV that actually dictates what the measurement outcome is which also means it has always had a definite, real property since its creation. This HV is currently inaccessible and beyond our human scope.
So, how can we test the EPR idea or the HV theory? In 1964, John S. Bell, an Irish Mathematician published an article demonstrating that measuring the spin of entangled particles could, in principle, serve as a decisive test for the existence of hidden variables. In the absence of hidden variables, the spin (or any property) of a particle would genuinely exist in a peculiar superposition state encompassing all potentialities. The initial experiments utilizing Bell’s ideas were carried out by John Clauser [Freedman, Stuart J.; Clauser, John F. (April 3, 1972). “Experimental Test of Local Hidden-Variable Theories”. Physical Review Letters. 28 (14): 938-941] at Columbia University in 1972. In his experiments Clauser employed photons and measured their “polarization.” Polarization is a directional physical property of light akin to the spin of an electron. His results strongly refuted the HV theory, thereby aligning with the QM perspective. In the coming years, physicist Alain Aspect carried out more sophisticated experiments that ruled out possible imperfections (loopholes) in Clauser’s initial experiments. The cumulative outcomes of these experimental studies not only enhanced the QM viewpoint but also unequivocally ruled out the hidden variables. Remarkably, J. Clauser and A. Aspect were two of the three experimental physicists honored with the Nobel Prize in Physics in 2022 for their groundbreaking investigations with entangled particles, persistently supporting quantum mechanics.
In contemplating the broader context outlined so far, we find ourselves compelled to acknowledge the inherently ambiguous nature of reality in the quantum realm. Particles exhibit properties that are in a superposition state leaving us only with uncertain claims and talking in terms of possibilities. This is equivalent to attributing wave-like properties. (For instance, a wave is extended and stretched and does not have a distinct position – it is in many places.) Yet, upon observation, the property becomes one of the possible realities and remains in that state. In other words, the wavy behavior goes away, and we now have a particle after the observation. This mind-blowing inference makes us ask what reality is. Is it a state of possibilities that only reveals itself only after we make an observation? Then, is it the act of observation that plays the critical role here? One is even tempted to say a reality exists solely through our perception. The interpretation of quantum mechanics is the topic of an ongoing debate. The experimental facts and the accurate mathematical description stay intact, but fundamental questions persist. Questions like “how does it make sense?”, “what does this all mean?” still temper with the minds of many philosophers and scientists. There are several interpretations of these quantum mechanical principles which we will only name here such as Many Worlds, Ensemble Interpretation, or Objective Collapse.
Beyond these various interpretations, a realization emerges from all this: our human understanding and knowledge remains fundamentally limited. Regardless of the advancements we make, there is a limit within the universe that puts us in a blind position. It is as if an imperceptible barrier restricts the boundary of human knowledge cautioning “no entry beyond this point.” It is as if “the keys of the unseen” is with God only (Qur’an 6:59), and that He creates every moment (Qur’an 55:29).
Another point to contemplate is the power of observation which in a sense showcases the significance of willpower. Our observations can change the reality in the quantum world. Extrapolating from this principle one may even argue that “if you really desire an outcome, visualize it, contemplate it, put in all your effort, and it will happen.” These are actually ideas that have widely been used by people in the field of self-improvement.
A third point is the wavy behavior we encounter in the quantum realm. An electron is like a wave until observation, after which point it becomes a “particle.” This prompts us to ponder: could the essence of everything be rooted in wave-like structures, suggesting a universe composed of foundational waves permeating all aspects of existence?
Note
- Nursi, Bediuzzaman Said. 2007. Al-Mathnawi al-Nuri (Seedbed of the Light), The Light Inc. NJ. p. 68. The Turkish original is “Nazar ve niyet mahiyet-i eşyayı tağyir eder.” “Nazar,” originally from Arabic, means eyesight, vision, viewpoint, perspective. We preferred to use “observation” here to make a better connection with the article. Already done with vision and from a viewpoint, “observation” is not a false translation.